A Clinical Study That Connects NAD+ and Aging
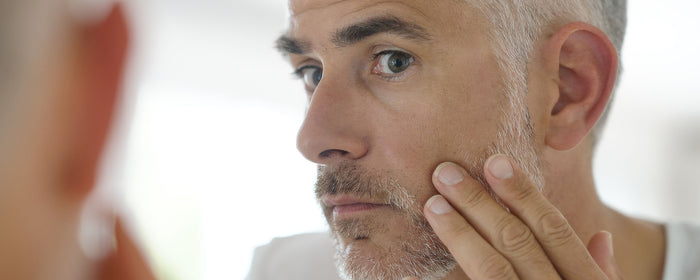
NAD+ is an enzyme aid that triggers cellular energy production for every living cell in your body. NAD+ primarily functions in our cell’s mitochondria, often nicknamed ”the powerhouse of the cell,” but it’s importance in our bodies was further highlighted by a group of scientists in Australia.
Hassina Massudi and a team of researchers from the University of New South Wales discovered that maintaining levels of NAD+ may play a role in aging.
The team drew their conclusions from studying NAD’s role in fueling the mechanisms needed to combat oxidative stress, a significant contributor to age-associated changes in the body.
What is oxidative stress?
Our mitochondria convert food into cellular energy but produce a terrible byproduct in its manufacturing process called free radicals. Free radicals can cause cellular damage, wreaking havoc on our cellular function.
Usually, our bodies have a healthy amount of antioxidants to combat the problem, keeping a delicate equilibrium of free radicals and antioxidants.
However, sometimes, we demand a little more energy from our bodies. We may overexert ourselves, kicking our mitochondria into overdrive. This overproduction of energy shifts the balance of our free radicals to an unmanageable level, known as oxidative stress.
Factors like intense exercise, lack of exercise, sleep deprivation, poor diet, drinking, smoking, and viral infections all lead to oxidative stress.
How does oxidative stress connect to aging?
In 1954, biochemist Dr. Denham Harman proposed the “Free Radical Theory of Aging,” hypothesizing that people age because of the imbalance of free radicals due to their role in damaging proteins, cell membranes, and DNA.
But later, in 2018, physiologist Dr. José Viña and his colleagues unveiled “A Free Radical Theory of Frailty,” arguing that free radicals do not necessarily dictate how long we live. Instead, Viña hypothesized the excess in free radicals causes frailty in older people, leading to a decline in their overall quality of life.
Whichever theory posits our understanding in the future, scientists universally agree that an excess of free radicals is a detriment to one’s health. And perhaps Viña’s approach more so questions how we define aging. Maintaining our wellbeing as we age is just as much a human desire as lengthening our lifespan.
Excitingly, the research from Massudi and the team in New South Wales shows a strong connection between the presence of NAD+ and oxidative stress, thus underlining NAD’s importance on how we age.
The study design.
The study obtained human skin samples from consenting patients scheduled for surgery at the Sydney Adventist Hospital in Australia. Participants included patients aged between 15-77 and newborn babies.
Researchers removed skin tissue from non-sun exposed areas of the pelvic region to study the least environmentally affected samples from their subjects.
The study monitored the following in correspondence with NAD+ to assess if NAD+ levels are linked to age-associated changes in our body:
1. Lipid peroxidation
Lipid peroxidation is a form of oxidative stress, specifically the degradation of lipids in our cell membranes.
2. Oxidative DNA damage
DNA is also vulnerable to oxidative stress. If the damage is left unfixed, our bodies can trigger cell death or mutagenesis, a process in which alters our genetic information.
3. PARP activity
PARPs or Poly (ADP-ribose) polymerase are a family of proteins involved in DNA repair, genomic stability, and programmed cell death.
4. Sirtuin 1 activity
Sirtuins, specifically SIRT1, are a class of proteins involved in regulating the cell.
The study results.
-
NAD+ levels declined with age in both males and females.
-
Lipid oxidation increased with age in males.
-
DNA damage correlated strongly with age in both males and females.
-
PARP activity significantly increased with age in males but was less evident in females.
-
SIRT1 activity negatively correlated with age in males.
Building on the study of aging.
The study developed by Massudi and the team provides quantitative evidence that the depletion of NAD+ may play a significant role in the aging process by limiting energy production, DNA repair, and genomic signaling.
Further study in the potential of boosting NAD+ with supplements or by natural means could unlock more curious findings. Already paving the road, a paper published in the Translational Medicine of Aging shows the therapeutic potential of boosting NAD+ as we age.
Whether solving the puzzle of oxidative stress will lead to a longer lifespan or better quality of life as we age, continued NAD+ research may redefine the boundaries of the aging process.